When you think that of electrical fields, you almost certainly consider electricity—the stuff that makes modern life possible, powering every part from household appliances to cell phones. Researchers have studied the principles of electricity because the seventeenth century. Benjamin Franklinfamous for his kite experiment, proved that lightning was indeed electrical.
Electricity has also enabled major advances in biology. A way called Electrophoresis allows scientists to investigate the molecules of life – DNA and proteins – by separating them based on their electrical charge. In addition to being commonly taught in secondary school biology, electrophoresis is a workhorse of many clinical and research laboratories. including mine.
I’m a Professor of Biomedical Engineering who works with miniaturized electrophoretic systems. Together with my students, my students are developing portable versions of those devices that quickly detect pathogens and help researchers combat them.
What is Electrophoresis?
Researchers discovered electrophoresis within the nineteenth century by applying an electrical voltage to clay particles and watching them move through a layer of sand. After further advances within the twentieth century, electrophoresis became the usual in laboratories.
To understand how electrophoresis works, we first need to clarify it electric fields. These are invisible forces that electrically charged particles comparable to protons and electrons exert on one another. For example, a particle with a positive electrical charge could be drawn to a particle with a negative charge. The law “opposites attract” applies here. Molecules can even have a charge; Whether it’s more positive or negative will depend on the variety of atoms that compose it.
During electrophoresis, an electrical field is created between two electrodes connected to an influence supply. One electrode is positively charged and the opposite negatively charged. They are positioned on opposite sides of a container crammed with water and a few salt, which might conduct electricity.
When charged molecules comparable to DNA and proteins are present in water, the electrodes between them create a force field that pushes the charged particles toward the oppositely charged electrode. This process is named electrophoretic migration.

Blanca H. Lapizco-Encinas, CC BY-SA
Researchers value electrophoresis since it is fast and versatile. Electrophoresis might help analyze several types of particles, from molecules to microbes. Additionally, electrophoresis might be performed using materials comparable to paper, gels, and thin tubes.
1972 physicist Stanislav Duchin and his colleagues observed one other variety of electrophoretic migration called nonlinear electrophoresis This could separate particles based not only on their electrical charge, but additionally on their size and shape.
Electric fields and pathogens
Further advances in electrophoresis have made it a useful gizmo for combating pathogens. In particular, the microfluidics revolution has enabled the emergence of tiny laboratories that allow researchers to quickly detect pathogens.
In 1999, researchers found that these tiny electrophoresis systems could do that too Separate intact pathogens by differences of their electrical charge. They placed a combination of several types of bacteria in a really thin glass capillary, which was then exposed to an electrical field. Some bacteria left the device faster than others attributable to their different electrical charges, allowing the microbes to be separated by type. By measuring their migration speed, scientists were in a position to discover each species of bacteria present within the sample in a process that took lower than 20 minutes.
Microfluidics has improved this process even further. Microfluidic devices are sufficiently small to slot in the palm of your hand. Their miniature size allows them to perform analyzes much faster than traditional laboratory equipment since the particles wouldn’t have to travel as far through the device for evaluation. This signifies that the molecules or pathogens researchers are in search of are easier to detect and fewer prone to be lost in evaluation.
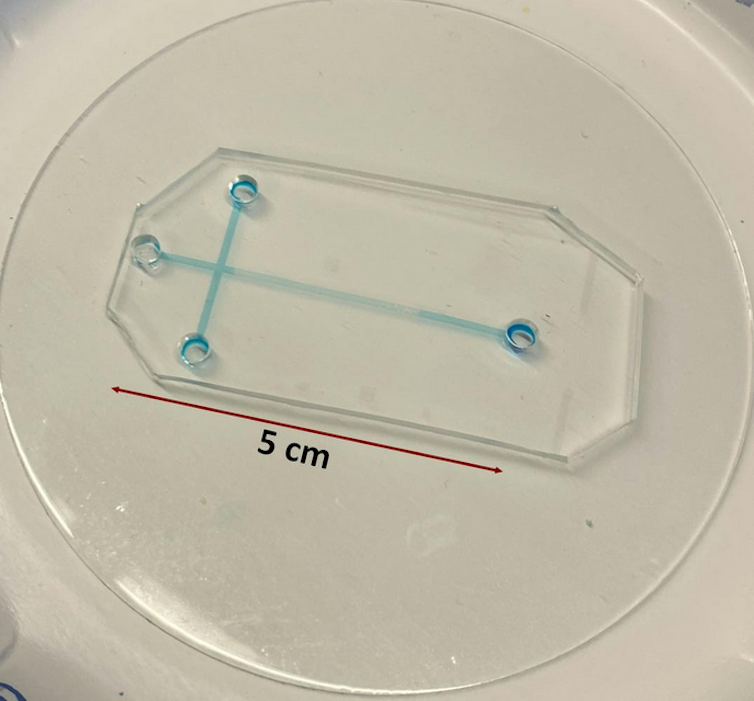
Alaleh Vaghef-Koodehi, CC BY-SA
For example, samples analyzed with traditional electrophoresis systems would must travel through capillary tubes roughly 11 to 31 inches (30 to 80 centimeters) long. Processing can take 40 to 50 minutes and shouldn’t be portable. In comparison, samples analyzed with tiny electrophoresis systems migrate through microchannels which are just one to five centimeters long. This means small, portable devices with evaluation times of approx two to a few minutes.
Nonlinear electrophoresis has enabled more powerful devices by allowing researchers to separate and detect pathogens based on their size and shape. My lab colleagues and I even have shown that combining nonlinear electrophoresis with microfluidics shouldn’t be only possible separate several types of bacterial cells but additionally live and dead bacterial cells.
Tiny electrophoresis systems in medicine
Microfluidic electrophoresis has the potential to be useful across industries. First and foremost, these small systems can replace traditional evaluation methods faster results, more convenience and lower costs.
For example when Testing the effectiveness of antibioticsThese tiny devices could help researchers quickly detect whether pathogens are dead after treatment. It could also help doctors resolve which drug is best for a patient by quickly distinguishing between normal bacteria and antibiotic-resistant bacteria.
My lab can also be working on developing microelectrophoresis systems to purify bacteriophage viruses that might be used for this purpose Treatment of bacterial infections.
As development continues, the facility of electrical fields and microfluidics can speed up how researchers can detect and combat pathogens.
image credit : theconversation.com
Leave a Reply